Studying stellar remnants allows us to find out how much gas and cosmic dust are ejected during a star’s lifetime. This will help us understand how successive generations of stars and planets are formed. We already know that elements produced by stars in the past gave rise to our Solar System, and has led to at least one planet rich in molecules and teeming with life.
The life-cycle of a star depends on its mass – more massive stars live faster and die younger than stars like our Sun. The pathways for massive stars and lower mass stars are shown in the image below.
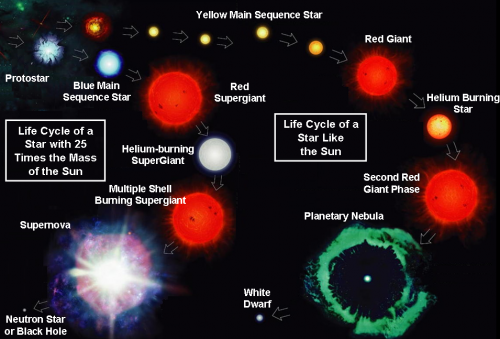
Low-mass stars
Stars less than about eight times the mass of the Sun live for a long time (100 million to 10 billion years). At the end of its current middle-aged period, the Sun will become a Red Giant star, expanding by a factor of a hundred with its outer layers extending out to the orbit of Mars! Such stars puff away their outer layers, leaving behind the hot, dense core of the star. We know that the core has a much smaller mass than the original star, so before they die stars actually lose most of their mass. This happens mostly in a period called the Asymptotic Giant Branch (AGB) phase, in which material is ejected in cycles, producing a series of shells around the star. Some examples are shown below. These multiple shells are effectively a fossil record of the star’s mass-loss history. About 90% of stars formed in our Galaxy go through this process, so understanding the evolution and mass loss from these stars is key to understanding how most stars live and die.
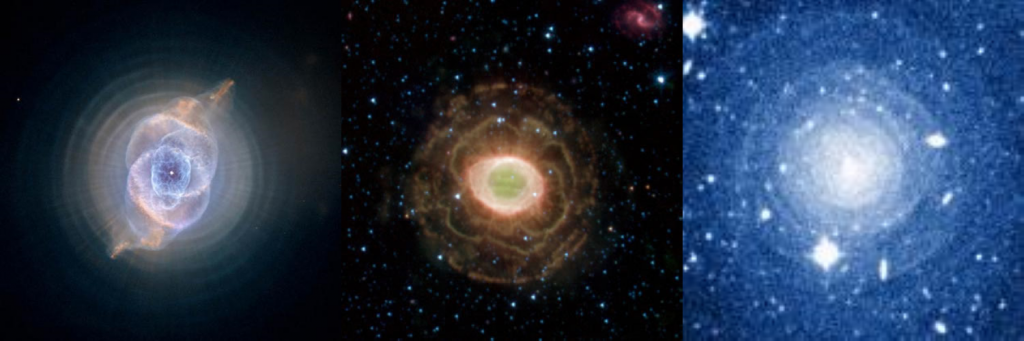
We know that the shells exist very far out from the star. But we don’t know how much of the star’s mass has been lost, because the shells are too cold and faint to have been detected by previous infrared telescopes. Herschel’s sensitive submillimetre camera, SPIRE, is perfectly suited to seeing the cold dusty shells, revealing exactly how much mass has been lost. These shells are also rich in atoms and molecules which emit light at specific wavelengths that depend on their chemical make-up, and which will be examined by Herschel’s spectrometers. Herschel will be particularly useful for looking at water molecules. Besides being important in the development of the conditions for life, water has a spectrum with many different features which are sensitive to the conditions the molecules are exposed to.
Other interesting compounds seen around dying stars include PAHs (Polycyclic Aromatic Hydrocarbons), made from hydrocarbon chains in a ring structure (see below inset), “bucky-ball” fullerene chain molecules, and amino acids, the building block of proteins. The PACS spectrometer will detect molecules like PAHs, and will also see the signatures of dust, including graphite, water ice, and CO2 ice. Besides telling us the compostion, this will tell us about the mass, temperature, and density of the gas, and what chemistry is going on. That will help to build a picture of how stars evolve, how much mass they eject, how they make dust, what chemicals exist in their environments, and how this material influences the formation of new stars and planets.
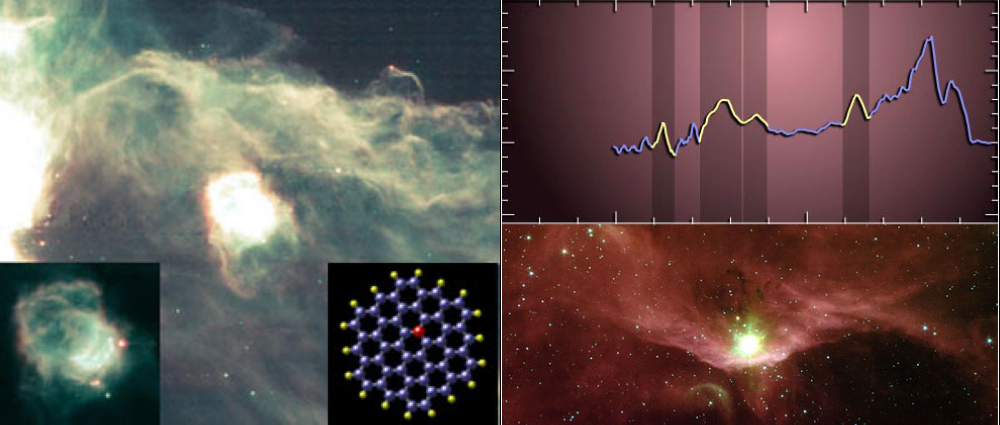
High-mass stars
Stars more than eight times heavier than the Sun also enrich galaxies with cosmic dust and heavy elements. Near the end of their relatively short lives they go through several phases in which they eject material in intense winds, sometimes with speeds up to 4 million MPH, and with huge amounts of ejected material. The star eta Carinae is thought to have lost over 80 times the mass of our Sun in the last 1,000 years in the form of gas and dust.
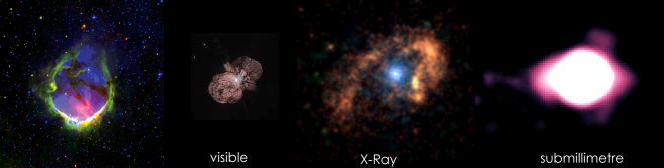
Massive stars eventually undergo supernova explosions, ejecting material outwards into the surroundings at fantastic speeds. The central core of the star is left behind as a neutron star or a black hole. How massive stars evolve before the supernova, and exactly how much mass they lose before they explode, are largely unknown. We need observations to find the colder ejected material to solve this problem, and which Herschel will provide. Astronomers have long wondered if the supernova itself may create cosmic dust. We have all heard the saying that “we are all stardust”, and new evidence suggests we could also be made from supernova dust. Observations of supernova remnants with NASA’s Spitzer Space Telescope (below, left) and the ground-based SCUBA camera (below, right) indicate amounts of dust ranging from 10,000 Earth masses up to the mass of our Sun in dust alone!
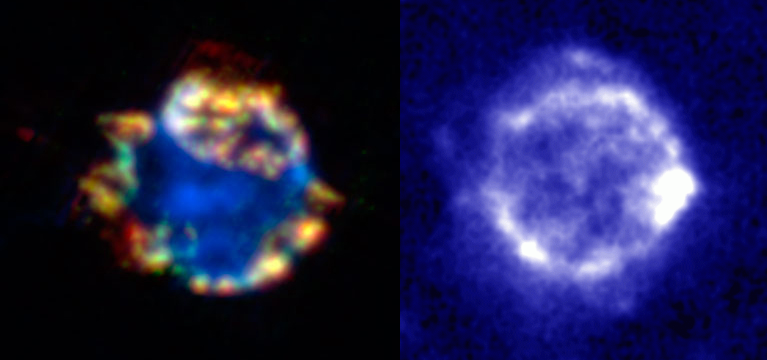
Herschel will look in detail at many evolved stars and supernova remnants. The PACS and SPIRE cameras will make images revealing the shells, to determine the mass-loss history and how much dust is created and puffed out of stars and supernovae. The HIFI and SPIRE-FTS spectrometers will find the signatures of molecules and dust and determine the environment and chemistry of the shells and supernova gas.